Proposed Research Agenda for Probabilistic Risk Assessment of Power System Reliability, Resiliency, & Adaptability to Improve Policymaking
This blog reviews current research & discusses the next steps in developing a comprehensive probabilistic risk assessment of power systems to inform policymaking.
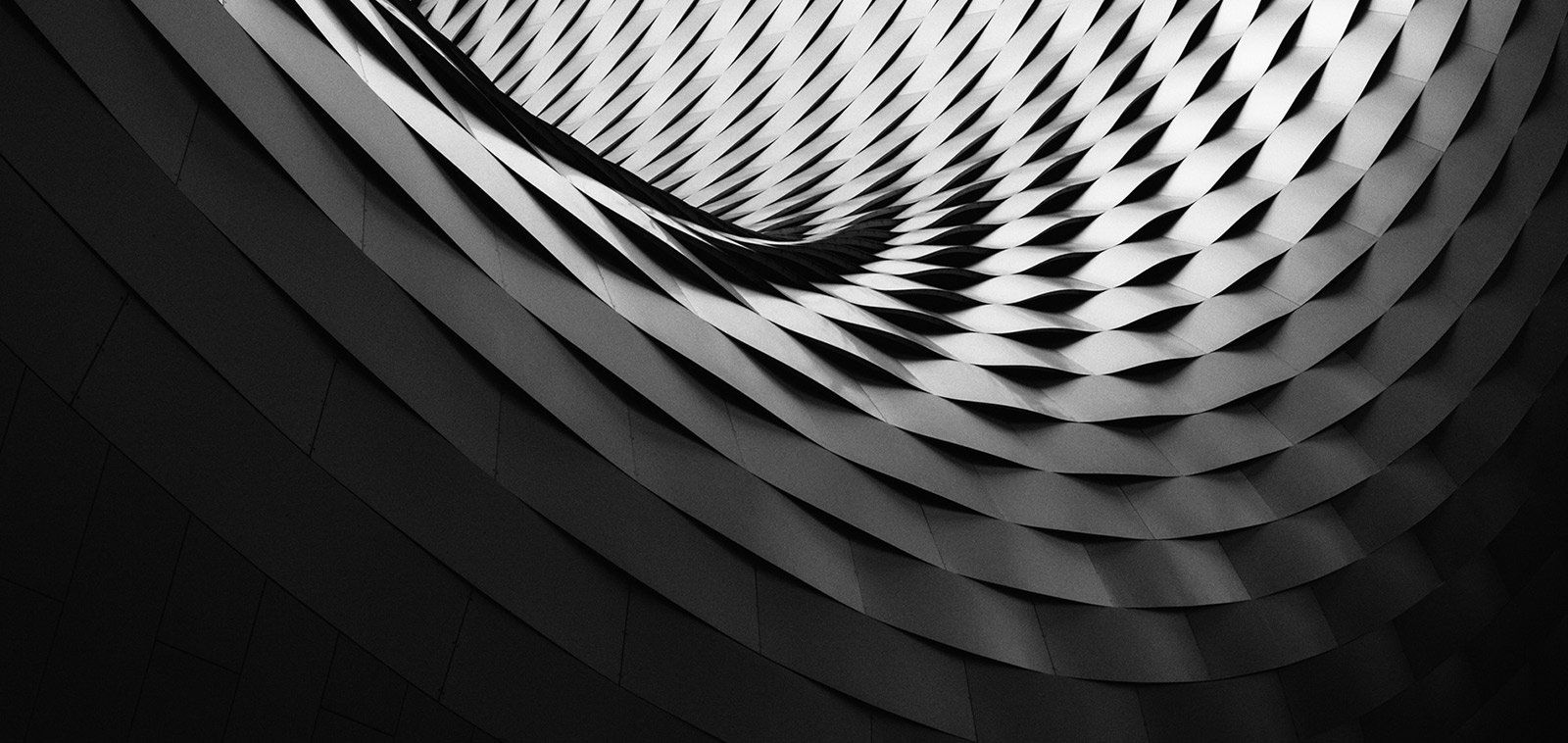
Introduction and Motivation
Improving power systems' reliability, resiliency, and adaptability (RRA) is an urgent priority. There is an overwhelming need for better models of the costs and benefits of policies and measures that compare the statistical distribution of existing and proposed solutions across affected populations (Felder et al. 2021). Given limited resources, the social and economic benefits of enhanced RRA need to be compared to the associated costs of doing so (NARUC 2023).
The rapidly changing context of the electric power sector is also increasing the need and urgency for improved RRA modeling to inform policymaking. The continued build-out of variable and intermittent renewables such as wind and solar PV, development and deployment of energy storage technologies, the occurrence of severe weather events and the changing climate, and increased electrification including electric vehicles (EVs), heating, and industrial processes all depend on reliable, resilient, and adaptable power systems.
Reliability refers to the power system's ability to function under typical conditions and contingencies without disconnecting firm load. It includes resource adequacy and operational reliability (historically called security). Resiliency refers to the ability of the power system to withstand severe but less frequent contingencies with minimal loss of load and the ability to restore service to all customers (FERC 2018) quickly. Adaptability refers to the ability of customers to mitigate the loss of electricity to reduce the costs of not having power (Felder and Petitet 2022).
Current Efforts to Improve Probabilistic Risk Assessments of Power Systems
Two significant ongoing efforts to improve the modeling capabilities to inform policymaking are resource adequacy modeling and resiliency modeling. Both can be subsumed under the umbrella term of probabilistic risk assessment of power systems. The research community has been actively developing and expanding these capabilities, focusing on reliability over the last several decades and, more recently, on resiliency.
Many textbooks codify these developments from resource adequacy to operational reliability (Li 2011, Billinton and Allan 2012, Li 2013, Li 2014, Brown 2017, Tuinema et al. 2020). The starting point was independent equipment failures using analytical techniques to calculate various adequacy metrics such as loss of load expectation (LOLE) and the amount of unserved energy (USE). The analyses expanded to include the transmission system, including interconnections and dependent outages, sometimes subdivided further to common-cause outages, component-group outages, station-originated outages, cascading outages, and environment-dependent failures (Li 2014). Other authors have used or are using different taxonomies. Wind and solar PV have spawned the concept of correlated events (ESIG 2021). Recent efforts focus on improving and expanding reliability metrics, using chronological models based upon Monte Carlo simulations, incorporating common-cause outages and correlated events, including flexible energy resources such as load, storage, and EVs, and improving transparency and transparency consistency of modeling efforts (ESIG 2021). An important topic in improving resource adequacy models relates to capacity requirements and mechanisms, both regulatory and market-based, with the rising penetration of wind and solar PV and falls under the question of what is the effective load-carrying capability (ELCC) of resources (Schlag 2020).
From the resiliency literature, one key concept is the resiliency trapezoid (Petitet et al. 2022). The trapezoid captures the duration and magnitude of an event but not the frequency and timing. These four factors affect an event's social and economic costs. An extended blackout due to severe cold weather is much more damaging than a slight load reduction during a short period on an afternoon with mild weather. The trapezoid points to the limitations of commonly used resource adequacy metrics such as LOLE, which have received much attention (e.g., Stenclik 2021) but the limitations of which have been well documented for decades (Felder, 2001).
Another strand in both literatures links actual power outages and their causes to models. Resource adequacy issues do not cause many power outages due to independent failure modes, as recent and long-standing references have noted (e.g., ESIG 2021, NARUC 2023, Billinton and Allan 2012 and their 1984 edition, Felder 2004). A cursory review of New York Times articles regarding power outages or examining U.S. Department of Energy data on system disturbances also supports this finding. Work in linking the state of the power system to its capabilities and failure modes is ongoing.
Proposed Research Agenda for Probabilistic Risk Assessment of Power Systems
The resource adequacy and resiliency literatures are converging under the umbrella of probabilistic risk assessment of power systems. As resource adequacy analyses expand the types of failure modes they address, extend their scope to include transmission, distribution, and load, and enhance the reliability metrics, they will arrive at a complete reliability and resiliency model of the power sector.
The elements identified below are needed to be incorporated within an analytical framework to provide policymakers with a comprehensive and detailed set of tradeoffs among the benefits and costs of various policies and measures to reliability, resiliency, and adaptability.
Additional Modeling Capabilities
1. Include operational reliability failure modes that lead to cascading failures, which have caused major blackouts.
2. Incorporate power system state dependent failure modes and capabilities into models.
3. Develop location-specific analyses (e.g., subregions, nodes/busbars) on the power system in addition to aggregate system-wide studies, which can be done given the flexibility and detailed capabilities of Monte Carlo techniques but require substantial computational efforts.
4. Present results to include uncertainty distributions in addition to expected values of costs, benefits, and other metrics.
Additional Policy Options
5. Add adaptability measures such as emergency shelters, backup power supplies, etc. since these measures mitigate the cost of power outages.
6. Determine public safety, health, and economic costs as a function based upon resiliency trapezoids to account for the timing, frequency, duration, and magnitude of power outages instead of relying upon the singular value of lost load (VOLL).
7. Evaluate multiple policy options such as regulatory mandates, incentives, and market mechanisms to improve accountability? for regulatory and market incentives given the strategic behavior of firms, organizations, and regulators.
Better and More Data
8. Acquire more comprehensive and detailed data sets of failure modes and power outages, especially due to dependent failures.
9. Incorporate Bayesian updating of data to account for non-stationarity of climate change and emerging performance of new technologies, which requires multi-year data collection.
References
Billinton, R., & Allan, R. N. (2012). Reliability assessment of large electric power systems. Springer Science & Business Media.
Brown, R. E. (2017). Electric power distribution reliability (Vol. 1). CRC press.
ESIG. (2021). Redefining Resource Adequacy for Modern Power Systems.
Felder, F. A. (2001). "An Island of Technicality in a Sea of Discretion": A Critique of Existing Electric Power Systems Reliability Analysis and Policy. The Electricity Journal, 14(3), 21-31.
Felder, F. A. (2004). Incorporating resource dynamics to determine generation adequacy levels in restructured bulk power systems. KIEE International Transactions on Power Engineering, 4(2), 100-105.
Felder, F. A., Unel, B., & Dvorkin, Y. (2021). Modeling strategic objectives and behavior in the transition of the energy Sector to inform policymaking. The Electricity Journal, 34(8), 107009.
Felder, F. A., & Petitet, M. (2022). Extending the reliability framework for electric power systems to include resiliency and adaptability. The Electricity Journal, 35(8), 107186.
FERC. (2018). 162 FERC ¶61,012. January 8.
Li, W. (2014). Risk assessment of power systems: models, methods, and applications. John Wiley & Sons.
Li, W. (2013). Reliability assessment of electric power systems using Monte Carlo methods. Springer Science & Business Media.
Li, W. (2011). Probabilistic transmission system planning. John Wiley & Sons.
NARUC. (2023). Energy Resilience Reference Guide. February 23.
Petitet, M., Felder, F., & Alhadhrami, K. (2022). One Year After the Texas Blackout: Lessons for Reliable and Resilient Power Systems (No. ks--2022-dp09).
Schlag, N., Ming, Z., Olson, A., Alagappan, L., Carron, B., Steinberger, K. and Jiang, H. (2020). "Capacity and Reliability Planning in the Era of Decarbonization: Practical Application of Effective Load Carrying Capability in Resource Adequacy," Energy and Environmental Economics, Inc., August.
Stenclik, D. (2021). Beyond 1-day-in-10-Years: Measuring Resource Adequacy for a Grid in Transition. ESIG Blog, November 29.
Tuinema, B. W., Rueda Torres, J. L., Stefanov, A., Gonzalez-Longatt, F., & van der Meijden, M. A. M. M. (2020). Probabilistic Reliability Analysis of Power Systems. Cham, Switzerland: Springer International Publishing.
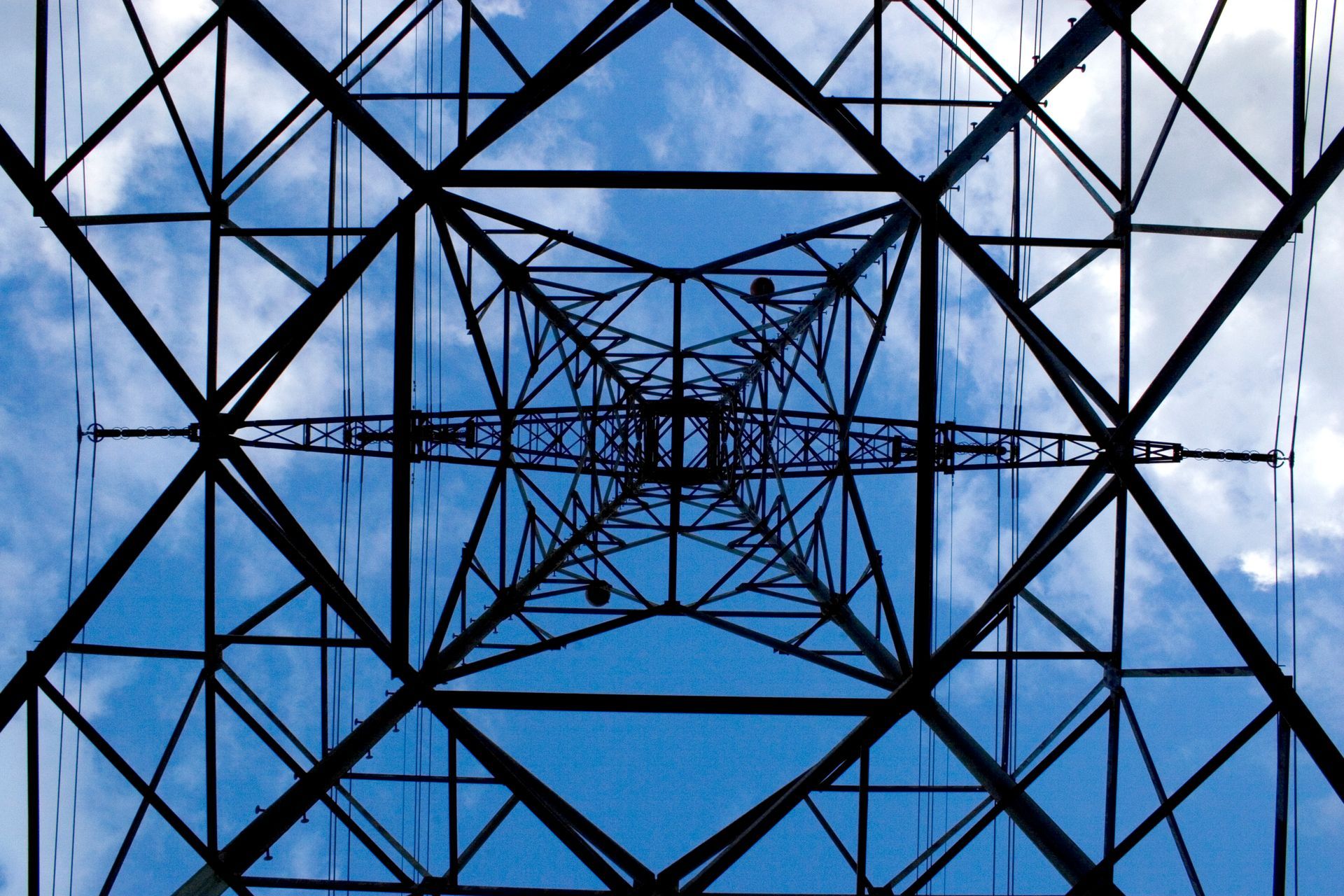